Prof. Dr. Markus Sauer | |
Telefon: | +49 931 31-88687 |
Since 2009 | Full Professor (W3), Chair of Biotechnology & Biophysics, Biocenter, University of Würzburg, Germany |
2003-2009 | Full Professor (C4), Chair of Applied Laser Physics & Laser Spectroscopy, Bielefeld University, Germany |
2008-2009 | Dean of the Physics Faculty, Bielefeld University, Germany |
2002 | Habilitation (Venia Legendi) in Physical Chemistry, University of Heidelberg, Germany |
1999 | Stay abroad, LBNL, Berkeley |
1997-2002 | Group Leader Biophysical Chemistry, Institute of Physical Chemistry, University of Heidelberg, Germany |
1995-1997 | Postdoctoral fellow, Institute of Physical Chemistry, University of Heidelberg, Germany |
1991-1995 | Dr. rer. nat. Physical-Chemistry, University of Heidelberg, Germany |
1985-1991 | Diplom degree Chemistry, University of Heidelberg, Saarbrücken, and Karlsruhe |
dSTORM provides virtually molecular structural resolution and quantitative information about the distribution of proteins in cells.
Imaging technologies are central platforms that drive fundamental research in virtually all disciplines across the biological and medical sciences. Visualizing cells and tissues by light and electron microscopy has led to more discoveries than any other technology in biological research. By seeing how a system looks, function can be understood and comparison made between healthy and unhealthy systems such as cells, tissue, a whole organism or population, plants, animals, and humans. In biology, nearly non-invasive fluorescence imaging techniques played a pivotal role in improving our understanding of cellular function. In medicine, different powerful techniques such as positron emission tomography (PET), x-ray computed tomography (CT), and magnetic resonance imaging (MRI) has provided insight into function and metabolism of organisms. This allows the investigation of the effectiveness of new targeted therapies down to the cellular level. However, our knowledge about the quantitative structural organization and interaction patterns of basic molecules and molecular machines indispensable to life is incomplete. Since detailed quantitative molecular information about targets of drugs and the biological consequences of their action is invaluable for designing new drugs and therapies, new refined imaging technologies for quantitative imaging with virtually molecular resolution are urgently required. However, obtaining high-resolution information about the architecture of cellular structures and their interrelations is hindered by the diffraction barrier of classical fluorescence microscopy. Super-resolution microscopy methods now can provide optical resolution that is well below the diffraction limit approaching virtually molecular resolution. They can be applied to biological samples and provide new and exciting views on the structural organization of cells and the dynamics of biomolecular assemblies on wide timescales. Especially, single-molecule based super-resolution fluorescence microscopy has the potential to fundamentally revolutionize our understanding of how nature encodes brain function at the molecular level. Among all powerful, high-resolution imaging techniques introduced in recent years including stimulated emission depletion (STED)1 and structured illumination microscopy (SIM)2, localization microscopy methods such as PALM3 and dSTORM4,5 excel because they deliver single-molecule information about molecular distributions, even giving absolute numbers of proteins present in subcellular compartments.6-8 This provides insight into biological systems at a molecular level that can yield direct experimental feedback for modeling the complexity of biological interactions.
Single-molecule localization microscopy methods such as dSTORM provide new techniques to visualize the distribution and quantify the absolute number of tumor-associated antigens on healthy and tumor cells. A paradigm shift is currently taking place in cancer medicine (hematology & oncology). It has been realized and clinical-proof-of concept has been obtained that immunologic anti-cancer treatments with antibodies and tumor-specific T cells are highly effective and indeed capable of curing advanced, end-stage malignancies that are resistant to conventional treatments. The spectrum of immune-based cancer treatments ranges from antibodies that bind to surface antigens on tumor cells to designer T cells that are genetically modified. In the first case bi-specific antibodies possessing two binding moieties are used, one for a tumor antigen and one that engages T cells or other immune cells to clear the tumor. For the latter approach, T cells are altered by gene transfer to express a synthetic chimeric antigen receptor (CAR) that binds to a surface antigen on tumor cells and is coupled to the killing machinery of the T cell. Several attributes make immunologic cancer treatments highly attractive, including their exquisite specificity for tumor cells due to recognition of antigens that are present on malignant but not on healthy tissues, thus sparing the toxic side effects of chemoradiotherapy. Moreover, an attraction of immunotherapy with CAR- and TCR(T cell receptor)-modified T cells is that a single administration may already confer tumor eradication and life-long protection from relapse, because T cells are ‚living drugs‘ that persist and proliferate until tumor clearance has been accomplished and form memory thereafter (see Center for Personalized Immunotherapy).
Another important attribute of immune based anti-cancer treatments is their extraordinary sensitivity. The detection threshold of CARs is in the order of less than 100 molecules of antigen per tumor cell, that of the TCR even lower. A critical challenge that arises in both research and clinical practice is that the sensitivity of antibodies and T cells is much higher than the sensitivity of detection methods such as immunohistochmistry (IHC) and flow cytometry (FACS) that are available to actually screen the patient‘s tumor for expression of the respective antigen. Here, single-molecule localization microscopy by dSTORM can be used advantageously to quantitatively map tumor-associated membrane receptors, ideally the entire tumor receptome, using commercially available fluorescently labeled antibodies (Fig. 1). This provides important information about possible tumor-specific surface targets for antibodies and T cells. That is, dSTORM imaging of the receptome of primary tumor cells enables the detection and selection of single or combinations of tumor antigens, and based on molecule density per cell, the selection of the optimal treatment modality for an individual patient.
Another biomedical field which can profit from the distinct single-molecule sensitivity of dSTORM is neurosciences. One of the major challenges in science is to understand the human brain and despite ongoing research it might be argued that our insights so far remain rather modest. Beginning with the identification of neurons as elementary building blocks of the nervous system, continuous technological advances in experimental resolving power have provided us with insights into signal transduction between neurons, which occurs at specialized neuronal sub-compartments, termed synapses. Synapse formation is a highly regulated process that requires the coordination of many cell biological events. Decades of research have identified a long list of molecular components involved in assembling a functioning synapse. Yet how the various steps, from transporting synaptic components to adhering synaptic partners and assembling the synaptic structure, are regulated and precisely executed during development and maintenance is still unclear. There is overwhelming evidence that defects in the synaptic function and in the fine structure of synapses are involved in the generation of severe neurological and psychiatric diseases. Here, again technical limitations hindered to date a better understanding of the structure-function relationships in the healthy and diseased brain. In a recent collaborative effort together with my colleagues Robert Kittel and Manfred Heckmann at the University of Würzburg, we introduced a novel procedure to determine endogenous protein numbers in tissue by dSTORM to analyze the molecular organization of the synaptic protein Bruchpilot and to estimate its copy number per presynaptic active zone in situ at the Drosophila neuromuscular junction (NMJ) (Fig. 2).8 We found that the active zone cytomatrix (CAZ) is composed of units containing ~ 137 Bruchpilot (Brp) proteins, three quarters of which are organized into about 15 heptameric clusters. Thus, single-molecule localization microscopy by dSTORM might pave the way for a refined understanding of the molecular organization of synapses and ultimately unravel how nature encodes function at the molecular level.9
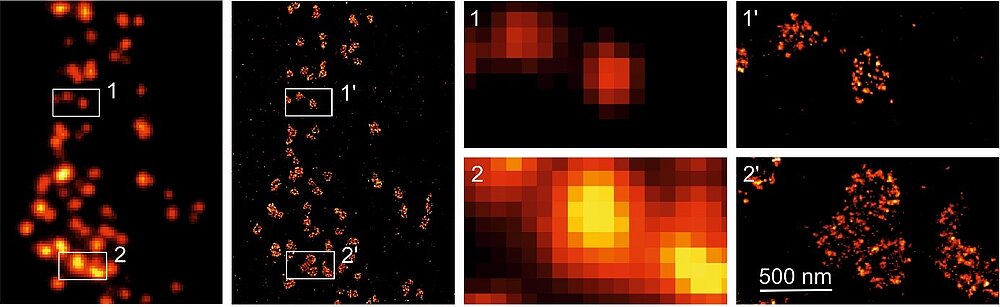
(1) Hell, S. W. & Wichmann, J. Opt. Lett. 19, 780-782 (1994).
(2) Gustafsson, M. G. J. Microscopy 198, 82-87 (2000).
(3) Betzig, E., et al. Science 313, 1642-1645 (2006).
(4) Heilemann, M. et al. Angew. Chem. Int. Ed. 47, 6172-6176 (2008).
(5) van de Linde, S. et al. Nat. Protocols (2011).
(6) Sauer, M. J. Cell Sci. 126, 3505-3513 (2013).
(7) Lando, D., et al. Open Biol 2, 120078 (2012).
(8) Ehmann, N., et al. Nat. Communications 5, 4650-4661(2014).
(9) Sauer, M, Heilemann, M. Chem. Reviews DOI:10.1021/acs.chemrev.6b00667